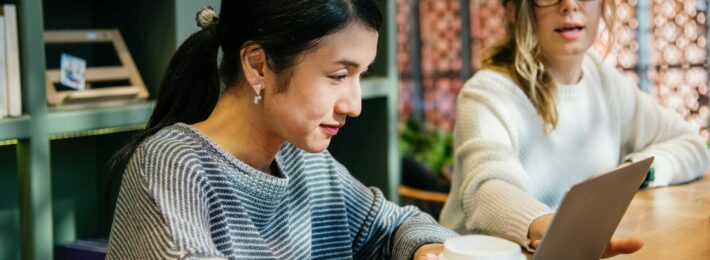
Establishing a Center of Excellence for Women in STEM has improved academic success, engagement, and retention of underrepresented, low-income, and first-generation undergraduate women in Bay Path University’s STEM majors. Here’s what Bay Path did, and some lessons learned that may be key if you would like to undertake a similar initiative.
by Gina Semprebon, PhD, Professor or Biology, Founding Director of the Center of Excellence for Women in STEM, Bay Path University; Christine Bacon, D.HEd, Associate Professor of Biology, Chair of Science, Bay Path University; and Lamis Jarvinen, PhD, Director of Partners for Scientific Inquiry
The Challenges We Faced
As seen in many other higher education institutions, our female students have become increasingly attracted to pursuing STEM majors over the past several years. At Bay Path University, a women-serving institution at the undergraduate level, we have responded by expanding our STEM offerings and have sought for effective ways to both attract and retain women in our STEM majors, as well as to serve as a networking and educational center for professional women in STEM fields in our region – many of the latter being our own graduates.
However, the typical academic challenges faced in gateway, foundational courses within the first two years of study have proven to be more challenging for students in recent years – particularly for our underrepresented and first-generation students – and especially in chemistry and math courses where quantitative literacy was imperative for success.
What We Learned and What We Did
We realized that we needed to update our curriculum and teaching methods. Fortunately, we discovered in our journey that we did not have to “reinvent the wheel,” as excellent national recommendations for building life science literacy are readily available, as well as a growing body of literature on how students best learn. In this article, we will share what we did and what we achieved – because your institution doesn’t need to reinvent the wheel either.
We undertook four steps to better attract and retain women in STEM:
- Leverage the research on how students best learn.
- Define a shared departmental vision.
- Develop an action plan with well-defined goals and objectives.
- Establish a Center of Excellence for Women in STEM to coordinate all efforts, obtain and administer funding, and assess outcomes (Figure 1).
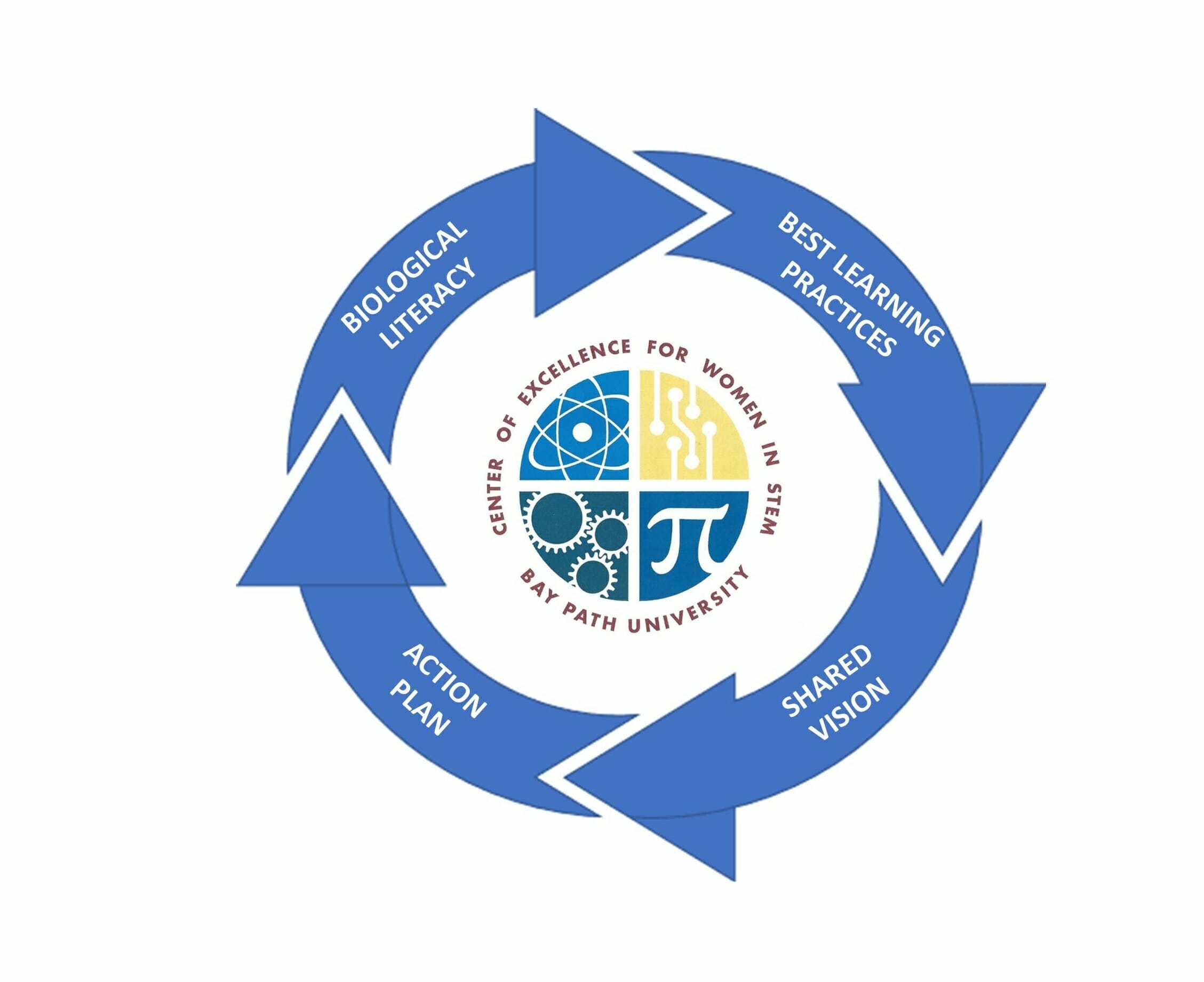
Figure 1. Our Pathway for Retaining Women in STEM
Step 1: Leverage current research on how students best learn
Synthesizing decades of research in the cognitive and developmental sciences, reports from the National Research Council (2000) emphasize the importance of transitioning student learning from content-focused to competency-focused. Armbruster et al. (2009) suggest offering students high-impact activities that are inquiry-based. They also point out that active learning and student-centered pedagogies have been demonstrated to improve both student performance and student attitudes of science majors and non-majors when compared to the more traditional approaches of the standard lecture or “canned” laboratory formats (e.g., Ebert-May et al., 1997; Hake, R., 1998; Bransford et al., 2000; Marbach-Ad et al., 2001; Udovic et al., 2002; Prince, M., 2004; Knight and Wood, 2005; Freeman et al., 2007; Preszler et al., 2007).
At Bay Path, we wanted to reduce the deficit of women majoring in science, technology, engineering and mathematics (Freeman et al, 2014). We also wanted to contribute to the growing number of women who choose to continue on and pursue careers in STEM fields. Drawing from the research on high-impact learning activities, we decided that providing students with meaningful, engaging, and high-impact learning experiences would improve retention and reduce academic failure rates in STEM courses (Freeman et al, 2014). We set out to learn as much as we could about these new pedagogical strategies in hopes of developing a realistic plan that would better serve undergraduate women at our institution, but next we needed a compass to guide and focus our efforts.
Step 2: Define a shared vision and framework for cultivating biological literacy
We found great inspiration and a very practical guide in the Vision and Change in Undergraduate Biology Education: A Call to Action Final Report (AAAS, 2011). This report set out recommendations deemed vital for cultivating high-impact practices for science students, and those recommendations provided us with an initial framework for restructuring our curriculum to focus more on the recommended central core concepts and competencies.
Our next task was to find a tenable way to implement these recommended approaches and high-impact practices. Again, fortunately, there was no need to reinvent the wheel. The Partnership for Undergraduate Life Science Education (PULSE) already exists, with the intent to catalyze the implementation of recommendations made in the 2011 report.
By joining the online PULSE Community and the PULSE New England Regional Network in our area, we were able to participate in both online and on-ground regional workshops designed to help us evaluate departmental strengths and weaknesses, develop a shared departmental vision, and establish well-defined action plans to help us to move forward in a coordinated and cogent fashion. PULSE served as a mechanism for educating ourselves regarding student-centered, active learning; strategic planning; and assessment techniques as we moved to act on the recommendations from the AAAS report.
The departmental shared vision we defined was aimed at improving the academic success, engagement, and retention of women in STEM with a particular focus on retaining low-income, minority, and first-generation college students because of the unique challenges these populations face.
Step 3: Determine Action Plan, Goals and Objectives
We crafted five general goals for implementing this shared vision:
- Provide early and pervasive exposure to undergraduate research by revising our current delivery system for providing research opportunities. Our current research endeavors were proving to be burdensome for our faculty and logistically unrealistic in terms of students’ availability to participate as well as the availability of the facilities and funding necessary to support such activities at a high level.
- Revise science and math offerings around student-centered, active learning pedagogies and provide faculty development opportunities to equip faculty with the tools to redesign and deliver courses this way.
- Enhance the effectiveness of mentoring opportunities – mostly in our gateway science and math courses and particularly for our first generation, low income and minority students.
- Provide funding that would reduce the need for our students to seek off-campus work and provide them with more time to engage in high-impact, on-campus enrichment activities.
- Expose students to meaningful role models by bringing in a variety of successful women in STEM fields.
How would we know our efforts were successful? We set these specific objectives:
- Redesign science and math courses and provide faculty professional development to implement active learning.
- Increase % of students who benefit from peer academic tutoring or mentoring support to at least 50%.
- Increase participation in research for upper level science majors to at least 30%.
- Increase participation in high-impact educational experiences to a minimum of 50%.
- Increase the 2-year retention rate for all 1st generation, minority, and low income full-time science majors by at least 25% above the current rate.
Step 4: Coordinate activities by centralizing different initiatives under a common umbrella
While this roadmap for revamping our science department and curriculum provided a great start, it quickly became apparent that more coordination would be necessary to ensure seamless delivery and assessment of our many new initiatives, implement our key strategies, reach our specific objectives, and overcome potential barriers to our success. To see how essential this coordination really was, take a look at Figure 2 (below) for a glimpse of the inherent complexity and multidimensional nature of this effort to redesign our department and curriculum. To achieve this, we had to establish a central, unifying hub to coordinate the different initiatives. This was our Center of Excellence for Women in STEM (CEWS).
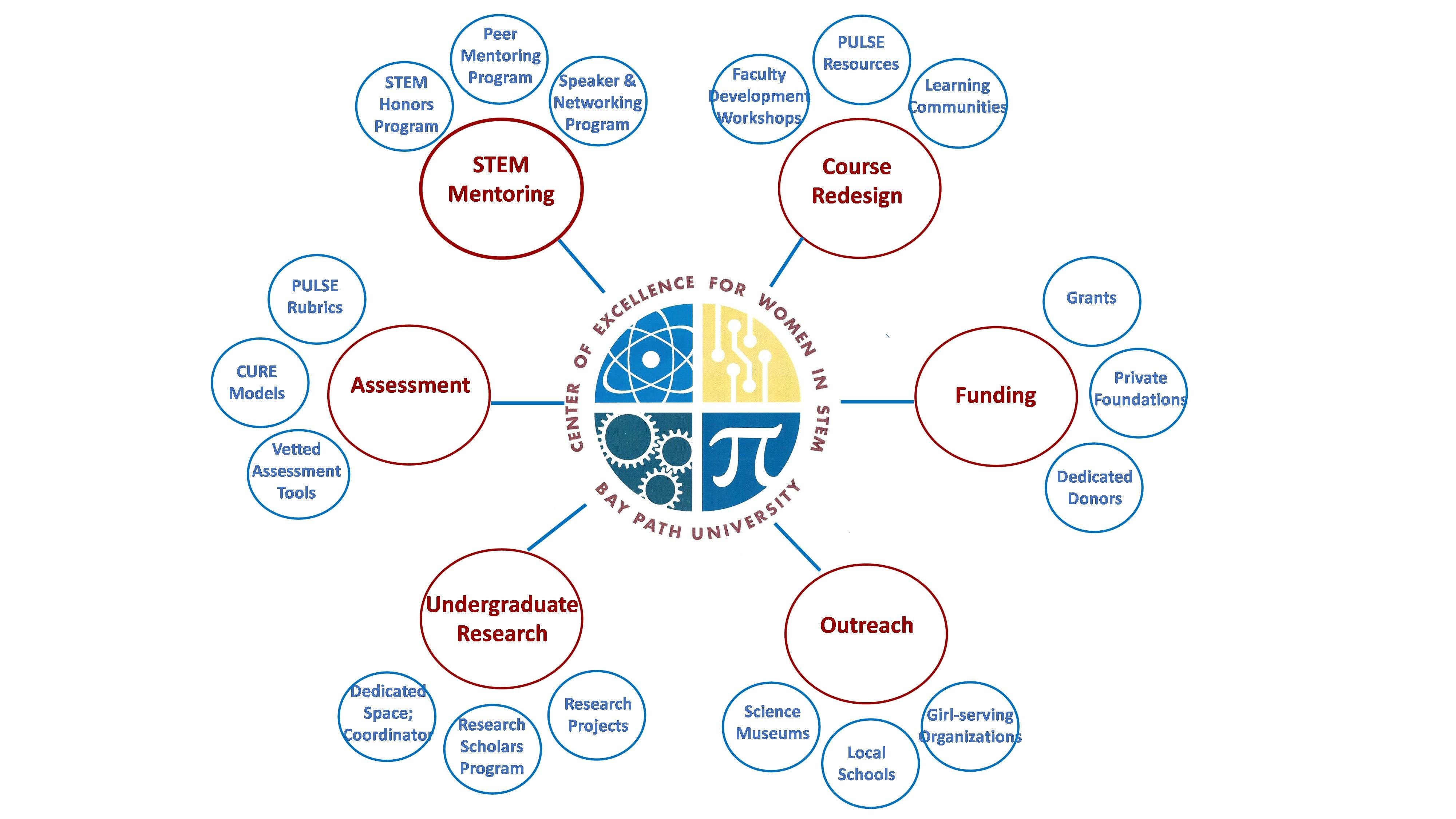
Figure 2. Key Components of Our Center of Excellence for Women in STEM
Inside the Center of Excellence for Women in STEM
Here is how CEWS has helped us obtain funding, undertake course redesign and faculty development, refocus our life sciences curriculum on core concepts and competencies, expand peer academic support and career exploration, and broaden student participation in high-impact learning experiences.
Obtaining funding
We obtained three National Science Foundation Grants aimed at attracting and retaining women in STEM (particularly low-income, first-generation, and minority women), and this helped us develop our Center of Excellence for Women in STEM. Then, two back-to-back S-STEM Scholarship grants provided a mechanism for assisting exemplary, low-income students with scholarship monies and peer tutoring, book cost assistance, and funding as paid research assistants. An NSF-IUSE grant aimed at improving our undergraduate science education provided funding for undergraduate research, high-impact learning activities, faculty development, and revising our curriculum for student-centered, active learning. In addition, we acquired advanced research equipment for undergraduate education and research via a Massachusetts Life Sciences Center grant.
Finally, we undertook an active campaign through CEWS to acquaint potential donors with the powerful impact these new departmental initiatives would have on student learning, retention, and success. This has led to ongoing, dedicated donor funding to support initiatives that we began as a result of grant support, including renovation of learning spaces and building dedicated research spaces.
Implementing course redesign and professional development for faculty
We needed to instill active, student-centered teaching and learning strategies in our science and math courses. We obtained a Title III grant from the Department of Education aimed at a campus-wide effort to revise our core curriculum along the tenets of student-centered, active learning principles; the grant also helped us develop a Teaching and Learning Center to assist faculty. We leveraged this grant to provide the extensive professional development necessary for redesigning both our core curriculum and departmental curriculum. Then, through CEWS, we developed faculty learning communities (e.g., a quantitative literacy learning community) to maintain our initial momentum and continue infusing new concepts and skills throughout our science and math curricula.
Integrating core concepts and competencies throughout the life science curriculum
We employed a complete set of rubrics available on the PULSE Community website as a diagnostic tool to evaluate the extent to which our department was implementing the recommendations of the Vision and Change (V&C) report (2011) as well as to map our science and math curricula. We found our faculty learning communities administered through CEWS extremely helpful for developing strategies to move our curricula and high-impact learning experiences closer to the V&C recommendations.
Expanding peer academic support and career exploration
We expanded the individualized peer academic support already available through our Academic Achievement Center in two ways. First, we instilled “peer lab mentors” in gateway courses that often pose the greatest challenge for students in their first two years. These peer mentors were not utilized simply as lab helpers to help the instructor set up and clean up after lab and mill around while students were working. Our peer lab mentors had very specific and expanded roles that proved to be pivotal to student success in these challenging classes.
While traditional peer tutoring is important, some of our students struggle to find time to go to our Academic Achievement Center because 1) they may have to work off-campus to afford college, or 2) they may be reticent to do so because of potential “labeling” by their peers as needing remedial support. By instead embedding peer lab assistants within the regularly scheduled lab period, we have been able to help students receive one-on-one assistance with the extensive and often challenging, embedded course-based research experiences (CURE); with lecture and lab assignments; with lab reports; and with review sessions that are built into the end of the lab period or are scheduled at convenient times for students who need to work and often virtually.
Second, we instituted major-specific peer mentors for the first-year class. These student mentors serve as a conduit for informal meetings, and as a means to personally invite first year students to participate in many enrichment events with their mentor (see the next section, below) as well as other high-impact experiences, such as peer-mentored student research projects. We trained these mentors and disseminated them through CEWS.
We also worked with our Career Office to provide career and networking events specifically designed for our science department and financed by CEWS.
Broadening student participation in high-impact learning experiences
We developed an array of high-impact educational experiences:
- Distinguished Speaker Series
- Brown Bag Lunch Seminars
- Women in STEM Networking Teas
- Journal Clubs
- Student Speaker Series
- Educational Field Trips
- Youth Outreach and Service Learning in STEM
- Early and Pervasive Research Opportunities (Course-based undergraduate research, Women in STEM Honors Program, Sterns Research Scholars Program)
- Regional Student Research Symposium and Poster Session
Importantly, through CEWS, we now track student participation in such events, allowing us to compare each activity against ethnicity, major, 1st generation status, class level, and Pell-eligibility status of our students. (For details, see “Barriers Overcome and Lessons Learned,” below.)
Our Results
Since we have formed our Center of Excellence for Women in STEM to administer and assess our STEM enrichment activities, we have already seen these results:
- We have redesigned 15 science and math courses for student-centered learning and to improve quantitative literacy.
- 100% of first-year and second-year students now benefit from peer mentor support.
- The 2-year retention rate for all first-generation, full-time science majors has increased from 51.5% to 78.1%.
- We instituted course-based undergraduate research (CURE) across all four years of our undergraduate science major curriculum (six courses) and within two of our non-major’s courses to enable a larger number of students to participate in authentic research experiences. This is vastly different from our traditional approach of providing independent research wherein a single faculty member serves as a mentor to a single student.
- We increased student participation in high-impact educational experiences well beyond our specific objective of at least 50%. In the past two years, 100% of first-year and sophomore students and 77% of juniors and seniors participate in course-based undergraduate research alone. Many additional students also benefit from individually-mentored research projects (around 12% of upper-level students and 4% of lower-level students). Consequently, last year:
- 93% of our students participated in authentic STEM research experiences.
- 73% of students presented their work as scientific posters and/or platform talks at an all-campus academic research symposium.
- 97% of students attended our distinguished Women in STEM speaker series.
- 70% of students participated in our departmental career mentoring initiatives.
- 57% of students engaged in STEM co-curricular and outreach opportunities.
- 76.9% of minority students were retained in the first two years, whereas, 79.3% of Pell-eligible were retained. Also, two-year retention rates for STEM students exceeded the retention rates of full-time students regardless of major by about 10%.
- In addition, we observed significant increases in student participation in extra academic help sessions outside of our lab extra help (first-generation student participation doubled, Pell-eligible student participation increased by 3.5 times and minority student participation increased by 186%).
- We also observed substantial increases in student participation from year one to year two in all high-impact enrichment activities by minority, first-generation and Pell-eligible students.
Barriers Overcome and Lessons Learned
Here is what we learned throughout the process of launching CEWS:
1. Faculty need institutional support.
While faculty are willing to try new pedagogical approaches, they need institutional support to allow them to feel comfortable in trying new approaches to teaching. This support can take the form of money and/or course-release, as well as substantial faculty development opportunities. Importantly, efforts by faculty to adopt new pedagogies also need to be valued on annual faculty evaluations (this was key for us at Bay Path). This comprehensive support – from workload to faculty development to faculty evaluation – provided the necessary blueprint and reward system for infusing student-centered active learning both in our core curriculum and our upper division science and math courses.
2. Faculty can be discouraged by the time commitment and workload involved.
Faculty recognized and supported the need for undergraduate research experiences but were discouraged by the extreme faculty time commitment required for individually-mentored research projects, the expense of running such projects, the lack of dedicated research space available for student research, and the faculty capacity or infrastructure needed to engage large numbers of students in individual research projects. These are real concerns. Supervising student research is generally not part of a faculty member’s teaching load and can require considerable hours and effort. The physical space required to do independent research can prove to be challenging when teaching laboratories are all that are available for students and faculty to engage in research projects. Also, engaging in undergraduate research can prove frustrating for both students and faculty due to disruption in availability of lab space and equipment.
We overcame these barriers by:
- Redesigning one of our teaching labs to be used as a dedicated research-only space.
- Hiring a Research Coordinator to assist faculty in overseeing the individually-mentored student research projects in this space.
- Instituting and assessing course-based undergraduate research experiences (CURE) early on and throughout all levels, through CEWS.
That third measure provided a mechanism by which an experienced faculty member could work with full-time faculty and adjuncts to conceive of and deliver projects, order and finance supplies, and assess outcomes. All CURE activities have proven to be highly successful in improving analytical skills, technical skills, increased content knowledge, perceived cohesion, project ownership, and science motivation. Improvement for students as they moved through each semester and from lower-level to upper-level course work is also clear.
3. It isn’t enough to put students in teams; we need to teach teamwork.
One big, consistent hurdle for our non-honors freshmen while working on authentic undergraduate research projects involves a lack of skill and some angst toward working effectively in groups toward a common goal. In practice, collaborative work has often led to a less than positive experience and has raised real frustrations around lack of conflict resolution, time management, and communication skills. Such frustrations undermine the well-documented benefits of collaborative and cooperative learning (e.g., Astin, 1997; Springer, Stanne, & Donovan, 1997; Tinto, 1998; Johnson, Johnson, & Stanne, 2000; Terenzini, Cabrera, Colbeck, Parente, & Bjorkland, 2001; National Survey of Student Engagement, 2006) and have the potential to derail efforts to produce high-performance teams and teamwork (Oakley et al., 2004).
To address this, we have now begun focusing more on training our faculty and peer mentors in techniques designed to build skills required for effective teamwork. We have found the guide by Oakley et al. (2004) to be quite helpful in providing explicit steps to us for training students on how to implement strategies for dealing with inevitable interpersonal issues and logistical pitfalls inherent in group work or serious scientific ventures. We have been encouraged to see improvement between the first and second semester of the first year, and the students are demonstrating good progress as they move from freshmen upward in their academic career.
4. Tracking student participation in high-impact learning activities reveals where we need to intervene.
By tracking student participation in high-impact learning activities, we were able to discover which activities are attended by different types of students, and conversely, which are not. First-generation college students (the first in their families to attend or complete college (Davis, 2010) and students of minority have a high rate of attendance at career advising events, academic field trips, and speaker events. However, activities and events that require students to participate in small groups wherein they would be required to interact (e.g., journal clubs, networking events), to present their own work publicly (e.g., student presentations of research or internship work), and to engage in STEM community outreach (e.g., after-school STEM enrichment for children) are not well-attended by either of these groups of students. Additionally, students in these categories do not generally seek out extra academic help (outside of the regularly scheduled help sessions).
Students in these categories appear to be less likely to self-advocate for such opportunities. This may be due to a number of reasons:
- They may simply not be aware of these opportunities.
- They may be aware of the opportunities but may not think that they can tap into them.
- They may not think that these extracurricular activities are of benefit to their overall educational experience.
- They may not have the benefit of their parents’ college experience to self-advocate for enrichment opportunities.
Our observations are supported by the work of others who have also investigated why first-generation and minority students may not be participating in or self-advocating for enrichment opportunities. One reason may be that they have a difficult time feeling that they belong to a community and therefore are less likely to feel entitled to opportunities that community has to offer (Davis, 2010). Because their parents did not attend or complete college, students may lack the know-how and experience to advocate and actively seek out opportunities; they may not feel that they have permission to ask for help and to assert their various rights (Horowitz, 2017). They may also be getting contradictory support from their parents who want them to attend class and do well but who do not understand the importance of extracurricular opportunities and community involvement (Baldwin, 2012).
In recognizing these patterns and potential challenges for students, we now realize that we need to be mindful of their cultural perspectives and experiences. We also need to be more proactive in reaching out to them and facilitating conversations that better explain how these high-impact activities support long-term achievement and success. We may also need to advocate on their behalf and begin an open dialogue with other colleagues in our university community so that they, too, can encourage students to engage in such activities. We believe we can further support all students by embedding outreach activities and non-traditional STEM experiences within the classroom requirements. This will be essential not only to help underrepresented women succeed in their STEM coursework but to help prepare them for success in STEM careers.
References
AAAS (2011). Vision and Change in Undergraduate Biology Education: A Call to Action. Washington, DC: AAAS. Available online at http:// visionandchange.org/finalreport/.
Armbruster, P., Patel, M., Johnson, E., Weiss, M., 2009. Active Learning and Student-centered Pedagogy Improve Student Attitudes and Performance in Introductory Biology. CBE—Life Sciences Education (8), 203–213.
Astin, A., 1993. What matters in college? Four critical years revisited. San Francisco: Jossey-Bass.
Baldwin, Amy. 2012. The first-generation college experience. Pearson Publication.
Bransford, J. D., Brown, A. L., and Cocking, R. R., 2000. How People Learn: Brain, Mind, Experience, and School. Committee on Developments in the Science of Learning. Washington, DC: National Academies Press.
Davis, J. 2010. The first-generation student experience. Sterling, VA: Stylus.
Ebert-May, D., Brewer, C., and Sylvester, A. (1997). Innovation in large lectures teaching for active learning. Bioscience 47, 601–607.
Freeman, S., O’Connor, E., Parks, J. W., Cunningham, M., Hurley, D., Haak, D., Dirks, C., and Wenderoth, M. P., 2007. Prescribed active learning increases performance in introductory biology. CBE Life Sci. Educ. 6, 132–139.
Freeman, S., Eddy S.L., McDonough, M., Smith, M.K., Okoroafor, N., Hordt, H., Wenderoth, M.P., 2014. Active learning increases student performance in science, engineering, and mathematics. PNAS 111 (23) 8410-15.
Johnson, D. W., Johnson, R. T., Stanne, M. E., 2000. Cooperative Learning Methods: A meta-analysis. University of Minnesota, Minneapolis: Cooperative Learning Center, http://www.co-operation.org/pages/cl-methods.html (accessed October 5, 2006).
Hake, R., 1998. Interactive engagement versus traditional methods: a six-thousand student survey of mechanics test data for introductory physics courses. Am. J. Phys. 66, 64–74.
Horowitz, Gail. 2017. First generation college students: How to recognize them and be their ally and advocate. Journal of College Teaching, Vol. 46(6), 8-9.
Knight, J. K., and Wood, W. B., 2005. Teaching more by lecturing less. Cell Biol. Educ. 4, 298–310.
Marbach-Ad, G., Seal, O., and Sokolove, P., 2001. Student attitudes and recommendations on active learning: a student-led survey gauging course effectiveness. J. Coll. Sci. Teach. 30, 434–438.
National Research Council. 2000. How People Learn: Brain, Mind, Experience, and School: Expanded Edition. Washington, DC: The National Academies Press. https://doi.org/10.17226/9853.
National Survey of Student Engagement Report. (2006). http://nsse.iub.edu/NSSE_2006_Annual_Report/docs/NSSE_2006_Annual_Report.pdf.
Oakley, B., Felder, R.M., Brent, R., Elhajj, I., 2004. Turning Student Groups into Effective Teams. Journal of Student Centered Learning, 2, (1), 9-34.
Preszler, R. W., Dawe, A., Shuster, C. B., and Shuster, M., 2007. Assessment of the effects of student response systems on student learning and attitudes over a broad range of biology courses. CBE Life Sci. Educ. 6, 29–41.
Prince, M., 2004. Does active learning work? A review of the research. J. Eng. Educ. 93, 223–231.
Springer, L., Stanne, M. E., Donovan, S., 2006. Effects of small-group learning on undergraduates in science, mathematics, engineering, and technology: A meta-analysis. National Institute for Science Education: Madison, WI, 1997; http://www.wcer.wisc.edu/ni se/CL1/CL/resource/R2.htm (accessed October 5, 2006).
Terenzini, P. T., Cabrera, A. F., Colbeck, C. L., Parente, J. M., Bjorklund, S. A., 2001. Collaborative learning vs. lecture/discussion: Students’ reported learning gains. J. Engr. Education, 90, 123-130.
Tinto, V., 1987. Leaving college: Rethinking the causes and cures of student attrition. Chicago: University of Chicago Press.
Udovic, D., Morris, D., Dickman, A., Postlethwait, J., and Wetherwax, P., 2002. Workshop biology: demonstrating the effectiveness of active learning in an introductory biology course. Bioscience 52, 272–281.